N2O formation on SCR catalysts at low temperatures
It’s not via nitrate decomposition for the standard SCR reaction
A summary of the article (click on the title for link)
“Dynamics of low temperature N2O formation under SCR reaction conditions over a Cu-SSZ-13 catalyst”
Xi, Y., Ottinger, N. A., Keturakis, C. J., Liu, Z. G. – Cummins Emission Solutions
Background
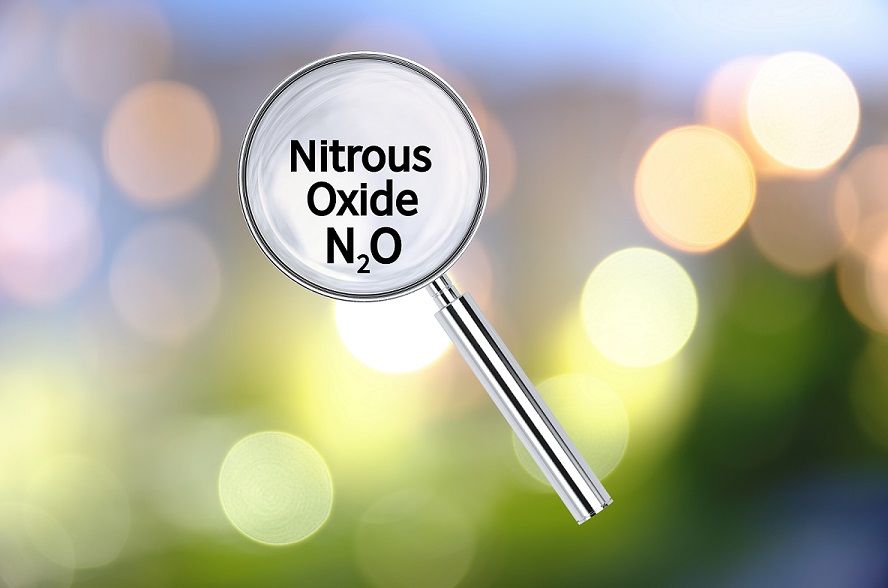
Search N2O and you will find that at room temperature, it is a “colorless non-flammable gas, with a pleasant, slightly sweet odor and taste”. It is commonly known as laughing gas due to “the euphoric effects of inhaling it” [1]. What’s not to like about it? But other than being popular for giving folks a high on the dentists chair, this gas is getting some pretty bad press. N2O is a potent global warming agent, almost 300 times as strong as CO2 [2]. Moreover, it is a stubborn molecule and stays in the atmosphere for more than a century (116 +/- 9 years, to be precise [3]). What are the major anthropogenic sources of N2O? Don’t say vehicular emissions just because this is a mobility article – the bigger culprit is agriculture and N2O emissions associated with fertilizers and manure are 4 times that from fossil fuels, and also the latter have been fairly constant or even decreasing since the 1980s despite an increase in road transportation [4]. Nevertheless, reducing N2O emissions from the vehicular sector is a high priority for regulators worldwide.
Modern diesel engines are equipped with SCR (selective catalytic reduction) technology, which is remarkably efficient at converting harmful NOx emissions to benign nitrogen. However, side reactions on the copper-zeolite catalysts also produce N2O in limited quantities. Like in the atmosphere, N2O is also resistant to destruction in the exhaust and to date, there are no catalysts which convert it to N2 once formed. The only option therefore, it to prevent formation in the first place. In the US, N2O emissions are currently limited for heavy-duty vehicles under the greenhouse gas regulations. Now, Europe is also considering adding limits in the upcoming Euro 7 legislation. Proposals suggest a limit between 10 – 30 mg/km for cars and vans and 160 mg/kWh for heavy-duty vehicles, possibly with a requirement to meet a combined limit along with another notorious GHG molecule, methane.
Researchers are looking at new catalysts and system architectures to prevent N2O formation, and are performing fundamental reaction kinetic studies to better understand the formation pathways. This article covers an example of the latter.
[1] RCSB
[2] EPA
[3] Prather, M. J. et al. Measuring and modeling the lifetime of nitrous oxide including its variability. J. Geophys. Res. D 120, 5693–5705 (2015).
[4] Tian, H., Xu, R., Canadell, J.G. et al. A comprehensive quantification of global nitrous oxide sources and sinks. Nature 586, 248–256 (2020). https://doi.org/10.1038/s41586-020-2780-0
Low temperature N2O formation via a “Cu-oxy” reaction intermediate
Urea is injected in the diesel exhaust stream which converts to ammonia before it enters the SCR catalyst. At temperatures below ~ 200 deg C, the SCR reaction is kinetically limited and instead the ammonia reacts with NOx to form ammonium nitrate (NH4NO3) deposits. These nitrates decompose to release N2O at low temperatures:
NH4NO3 –> N2O + 2 H2O
The above, however, is true only when NO2 is involved as a reactant through the so-called “fast-SCR” reaction. In fact, under standard SCR conditions which include NO only, the authors show that N2O can be formed at temperatures down to ~ 150 C, which is not supported by the decomposition route for ammonium nitrates as these are rather stable at temperatures below 200 C. This study proposes an alternative pathway for N2O formation at such low temperatures for the standard SCR reaction.
How can we be sure that nitrates are not involved in N2O formation during standard SCR ?
(1) In a transient experiment where the catalyst was first treated with NH3 + O2, N2O formation was found to begin as soon as NO was introduced. The prompt N2O formation suggests that there was no intermediate nitrate formation which would have resulted in a lag before which N2O was detected.
(2) Temperature programmed desorption (TPD) experiments in which NO, NO2 and a combination of NO2 + NH3 were adsorbed on the catalyst showed that N2O was released only for the last case of adsorption with NO2 and NH3, and the release occurred in the 250 – 350 C temperature range. This combined with a peak of NO2 release in the same temperature range showed that ammonium nitrates formed require NO2 and NH3, and the decomposition occurs at T > 250 C. Again, this cannot explain the N2O formation observed as low as 150 C during standard SCR mentioned above.
To explore the chemistry involving standard SCR, titration experiments were done where the catalyst was pre-treated with various combination of O2, NO, and NH3. This was next followed by exposure to NH3 and NO in the absence of O2. It was found that NO consumption was proportional to the extent of oxidizing environment in the pre-treatment step, and the N2O formation was in turn proportional to the NO consumption. It was therefore proposed that a “Cu-oxy” species participates as an intermediate reactant for N2O formation. When the concentration of NO is increased in the feed during SCR at 200 C, the N2O formation was found to exhibit a maximum vs. the NO concentration. The authors explain that the initial increase is associated with NO availability being the rate limiter, while the decrease is associated with the density of Cu-oxy species being the rate limiter.
The final reaction mechanism looks as follows (this is not balanced, and is meant to show the main pathway – see the paper for full details on the Cu-oxy species):
[Cu-oxy] + NO + NH3 –> N2 + H2O + Cu
[Cu-oxy] + NO + NH3 –> N2O + N2 + H2O + Cu
So what ?
Diesels are expected to remain the mainstay for goods transportation and off-road machinery for several years. Engine manufacturers will have to continue to adopt advanced after-treatment systems and seek improvements in deNOx solutions for meeting the upcoming standards. It takes a few years for fundamental studies such as these to translate into improved commercial catalysts. It can be expected that armed with this new mechanistic insight, engineers can further improve engine calibration and catalyst formulations and mitigate the formation of N2O. The ability to control N2O can also enable greater reductions of NOx, due to the NOx-N2O trade-off that is seen on commercial catalysts. Finally, it should be noted that while this study focused on Cu-zeolites, there are other classes of SCR catalysts which can lower the N2O formation and likely have a different mechanism.
As is often the case with research, this will hopefully also spur further studies on this important topic.
Other recent posts
China Stage 4 Fuel Consumption Standard for Light Commercial Vehicles
A summary of Chinese Stage 4 fuel consumption standards for light commercial vehicles.
Mobility in India – excerpts of recent headlines
A summary of reports from a couple of leading newspapers in India, taken during end of Sept to early October, 2024 points to the rapidly changing mobility and energy landscape in India.
Biofuels summit in Washington DC highlights role of ethanol in transport decarbonization
The biofuels summit hosted by Growth Energy at Washington DC highlighted the role that ethanol continues to play in decarbonizing the transport sector.
Like it ? Share it !